Preparation
and Characterization of Linseed Oil Based Nanoemulsion for Transdermal Delivery
of Losartan
Atanu
Sinhamahapatra*1, Ishita
Singha2, Nibedita Pati3
Dr. B.C.Roy College of Pharmacy & AHS. Durgapur, West
Bengal, India.
Correspondence:
atanusinha02@gmail.com
Abstract
Conventional delivery of losartan
through oral route suffers from very low bioavailability (~38%). In the present
work, we have explored the efficacy of nanoemulsion system for consisting of
linseed oil, tween 80, span 80 (2:1) and water for transdermal delivery of
losartan. While the average droplet size of the nanoemulsion lied in a range of
287 to 350 nm, zeta potential of -50mV was recorded. Exhaustive stability tests
on the formulation containing the maximum entrapped drug (~78%) revealed that
the nanoemulsion was stable both under conditions of stress, and in long term
storage. The pH of formulation was recorded to be 5.38± 0.01 and was considered
fit for transdermal applications. A low permeation flux through porcine skin of
1.39 μg/cm2/h from the optimized formulation revealed that the
nanoemulsion is an ideal carrier for delivery of losartan in lengthy
therapeutic schedules.
Keywords: nanoemulsion; linseed oil;
transdermal; losartan
INTRODUCTION:
The skin is the largest organ in
the body and has a surface area of about 1.5 to 2 mm2, hence the transdermal
route providing most promising and favorable route and an area of attraction
for the researchers [1]. The limitation of oral route can be successfully
overcome by transdermal delivery.
Besides more convenient and easy administration it also avoids the
hepatic metabolism and gastrointestinal intolerance, and also immediate
withdrawal is possible of the drug if necessary [2]. The limitations of
transdermal drug delivery are principally associated with the barrier function
of skin due to the present of stratum corneum as the
drug molecules cannot readily pass through this impervious barrier [3]. Many
works in the recent years have intended to design topical vehicle for
controlled and on demand modification of drug permeation through the skin.
Nanoemulsion plays a role as a vehicle for delivery [4]. The mechanism may
involve in enhancing permeation through permeation enhancer which may present
as ingredients and help reducing the diffusion barrier of stratum [5, 6].
However, such permeation enhancers and solvents are known to cause undesired
effect on the skin upon prolonged use. Transdermal permeation can be improved
by nano emulsion.
Nanoemulsion can be defined as
o/w emulsions with mean droplet diameters ranging from 50-1000 nm, and are
transparent thermodynamically stable and translucent dispersion and oil and
water [7].Its contain a mixture of oil, surfactant, co-surfactant and an
aqueous phase. Losartan is a model choice of drug for our study act as
angiotensin II receptor antagonist widely used as an antihypertensive drug [8].
Oral administration of losartan is most common and traditionally use but in
these route bioavailability is only 33% and significantly first pass metabolism
occurs and the drug have very low molecular weight (461.01 Da) and also
containing log P 4.5 with very short biological half-life (2h) [9,10]. Due to
these properties it became more flexible for transdermal delivery.
Biocompatible nanoemulsion improves the solubility and stability of the
encapsulated drug [11].
Our main objective of the study
is to develop and characterize linseed oil based nanoemulsion for transdermal
delivery of losartan. A pseudo-ternary phase diagram was plotted in order to
identify the self-emulsifying region, from which the most stable formulation
was selected for further evaluations.
MATERIALS
AND METHODS
Materials
Losartan potassium was obtained
as a gift from the HOD, Department of Pharmaceutics, Dr. B.C. Roy College of
Pharmacy & A.H.S., Durgapur (India). Tween 80 (sorbitan
monolaurate) and Span 80 (sorbitan
monooleate) were purchased from Loba
Chemie (Mumbai, India). Linseed oil was obtained from
Shiv Sales Corporation (New Delhi, India). All reagents used in the study were
of analytical grade. Double distilled water obtained from laboratory
purification systems was used for all experiments.
Construction of pseudo-ternary
phase diagram (PTD):
The PTD was constructed using
aqueous titration method as described by Malakar et
al., 2014 [12]. The surfactant system (Smix) was
constituted by Span 80 and Tween 80 in a fixed proportion of 1:2. Linseed oil
was the oil phase constituent, and aqueous phase was composed of freshly
prepared double distilled water. Initially surfactants were mixed with oily
phase in ratios ranging from 2:8 to 8:2 and was then titrated with water. After
each titration, samples were subjected to high sheer homogenization (Bharat motors,
India) at 8000 rpm for 10 min at room temperature. Each samples were inspected
visually for clarity and phase homogeneity. Clear and transparent formulations
identified as nanoemulsions were subsequently marked
in the phase diagram using Microcal Origin 6.0 (Microcal Software, Inc., USA) to identify the nanoemulsion
zone.
Preparation of nanoemulsion:
Nanoemulsion formulations
selected from the constructed phase diagram were prepared according to the
composition illustrated in Table 1. 10 mg of the drug was dissolved in linseed
oil for each selected formulation. Smix was then
added in an appropriate ratio and the mixture was equilibrated under magnetic
stirring for 15 min. Water was subsequently added drop-wise with continuous
stirring. The primary coarse emulsion thus formed, were further subjected to
high sheer homogenization at a temperature under 20 °C.
Physicochemical characterization
of nanoemulsion:
Mean globule size and polydispersity index (PDI) of the formulation was recorded
using a Zetasizer Nano ZS series (Malvern
Instruments, UK) equipped with a 4mW He-Ne laser (λ= 633 nm). Samples
diluted 1000 folds were placed in the module and data were recorded in
triplicates for each batch at 25 °C. Zeta potential analyses were performed by
measuring the electrophoretic mobility using the same instrument after dilution
of the samples in 10 mL water.
pH values of the nanoemulsion
were recorded at 25 °C by directly immersing the electrode of a calibrated pH
meter into the undiluted formulations.
Drug entrapment efficacy:
Losartan containing nanoemulsions were first exposed to centrifugation at
14,000 rpm for 20 mins in Remi
cooling centrifuge (Remi, India) to separate the
un-entrapped drug from the final formulation. 100 µL of the supernatant was
diluted with methanol and the concentration of the drug was quantified through
UV-Vis spectroscopy.
Stability studies:
Different formulations were
examined for their resistance to centrifugation stress. Aliquots of samples (10
mL) were subjected to centrifugation at 6,000 rpm for 20 min and observed for
any evidence of creaming, cracking or phase separation.
Freeze-thaw stability of
nanoemulsion was determined by exposing the formulation to three freeze-thaw
cycles, which included freezing to –10 °C for 24 h in a freezer followed by
thawing at 40 °C for 24 h. The formulation was then evaluated for particle size
and zeta potential.
Shelf life stability study was
conducted by storing the formulations at room temperature for 3 months. Various
physical parameters such as clarity, phase separation, creaming, creaking,
color, and odor were observed. Similarly, droplet size, zeta potential and pH
were recorded. Only the formulations which complied the stability tests were
considered for further studies.
Quantification of losartan
The quantification of losartan
was performed using a Shimadzu 1800 UV-Vis spectrophotometer (Shimadzu, Japan).
An absorbance (y) vs. concentration (x) plot, y=0.455x – 0.0134, R2= 0.998, was
first generated and applied for losartan estimation throughout.
Preparation of skin
Goat ear skin for permeation
studies was obtained from a local slaughter house. Then the hair were removed
using an animal hair clipper and subsequently, full thickness of the skin was
harvested. The fatty layer adhering to the dermal side was removed by surgical
scalpel. The skin were washed with 50 mM PBS
(phosphate buffer saline, pH 7.4), and stored at -20 °C until further use.
Ex vivo permeation studies
Ex vivo skin permeation study of
the selected formulation was carried out using a vertical Franz diffusion cell
with an effective diffusional area of 1.64 cm2 and receptor compartment
capacity of 90 mL. The excised skin was fixed between
the donor and receiver compartment with stratum corneum
side facing the donor compartment. The receiver compartment was filled with PBS
which was stirred with a magnetic rotor at 500 rpm, and the entire assembly was
placed in an incubator to maintain a temperature of 37± 0.5 °C. The skin was
initially allowed to equilibrate for a period of 1 hr. 2 mL of nanoemulsion was then introduced into the donor compartment
and sealed with aluminum foil to prevent evaporation of water. 5 mL of samples
were withdrawn at regular intervals and replaced by the same amount of PBS. The
samples were filtered and amount of drug permeated was quantified using a
UV-Vis spectrophotometer, by measuring the absorbance at 254 nm wavelength.
Permeation flux
The amount of drug (Q) from the
nanoemulsion permeated through excised skin was plotted against the function of
time. The slope and intercept of the linear portion of plots were obtained
through regression method. The steady state was calculated from the slope
divided by the effective diffusional area [12, 13]
Jss= (dQ/ dt)ss. 1/A
Where Jss
is the steady-state permeation flux (µg cm-2 h-1), A is the area of skin (cm2),
(dQ/ dt)ss
is amount of drug passing through the skin per unit time at a steady state.
2.10 Statistical Analysis
All experiments were performed in
triplicates using freshly processed sample and the results were reported as
mean± standard deviations.
RESULT
AND DISCUSSION
Pseudo ternary phase diagram
(PTD)
Non-ionic surfactants such as
Tween 80 and Span 80 were chosen for the formulation of linseed oil based nanoemulsions according to their HLB values and phase behaviour. The flexibility of surfactant film, its affinity
for water, and the interfacial tension are important parameters involved in the
formation of nanoemulsions. In this aspect, the
construction
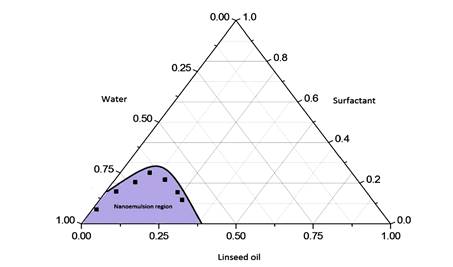
Figure 1: Pseudo-ternary phase
diagram of linseed oil, surfactant (Span80 and Tween 80 in ratio of 1:2) and
aqueous phase. The darkened region signifies the nanoemulsion zone.
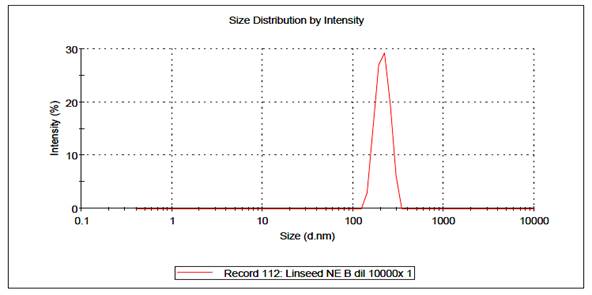
Figure 2: Droplet size
distribution of losartan loaded nanoemulsion.
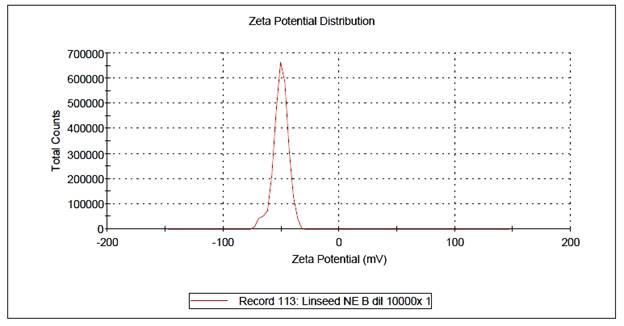
Figure 3: Zeta potential analysis
of losartan loaded nanoemulsion (F5).
Table 1: Physico-chemical parameters for different nanoemulsion
formulations
Codes
|
Average
Droplet size(nm)
|
Polydispersity index
|
pH
|
Zeta
potential (mV)
|
Drug
entrapment (%)
|
F1
|
287.38±
3.4
|
0.35
|
4.60±
0.4
|
-38
|
72±0.7
|
F2
|
298.41±
3.61
|
0.41
|
4.64±
0.12
|
-40
|
73±
0.72
|
F3
|
297.42±
3.49
|
0.38
|
5.20±
0.38
|
-42
|
74±
0.8
|
F4
|
299.01±
3.7
|
0.34
|
4.81±
0.12
|
-51
|
74±
0.79
|
F5
|
300.02±
3.75
|
0.37
|
5.38±
0.01
|
-50
|
78±
0.87
|
F6
|
320.34±
3.82
|
0.39
|
5.05±
0.24
|
-48
|
75±
0.79
|
F7
|
350.73±
3.91
|
0.42
|
6.2±
0.32
|
-47
|
76±
0.81
|
*Results are expressed as mean±
S.D.
of PTD has been widely employed
to understand the balance among mixtures of surfactants, oil and water in the
emulsion systems. In our work, PTD was constructed based on macroscopic
observations of different type of dispersion obtained from mixtures produced.
We used the aqueous titration method at room temperature (25±2 °C). Several
sorts of dispersions, including conventional emulsions and nanoemulsions
could be observed with the mixture of surfactants used. We have observed that
the formation of a single-phase region at very low oil concentrations and high
surfactants concentrations. The formation of a milky single-phase system in
most part of the diagram suggests that the mixture of surfactants was able to
minimize the surface tension between aqueous and oily phase, thus promoting the
formation of conventional liquid emulsions.
Figure 4: Linseed oil nanoemulsions before and after long term stability
experiments
Pseudo-ternary phase diagrams for the primary nanoemulsions were developed so that nanophasic
regions could be identified for the optimization of formulations. From the
phase diagram, it was clear that Smix 1:2 was
significantly reduced the interfacial tension on the w/o interface and a
considerable self-emulsifying area appeared in the phase map. From the samples
F1 to F7 we selected the optimum on the basis of average droplet size and drug
entrapment. Among them, F5 contained the maximum drug entrapment in where the
particle size is within range. The optimum concentration of oil phase and
surfactant that was selected for the development of o/w nanoemulsion was 16.2 %
and 21% respectively.
Nanoemulsions were characterized on the basis
average droplet, size polydispersity index, pH, zeta
potential, and percentage of drug entrapment.
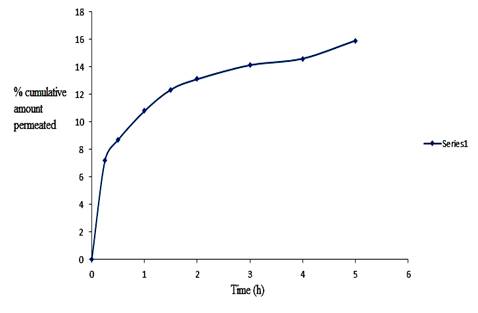
Figure 5: Ex vivo permeation of losartan from
nanoemulsion at 37 °C.
And the value which was obtained
was shown in Table 2. Texture and stability of the nanoemulsion depends on the
distribution of droplet size is well established [14]. Dynamic light scattering
was used to measurement the droplet size of the formulations, were shown in the
table 2. The average droplet size of the nanoemulsion lied in a range of 287 to
350 nm. Polydispersity index is assessing of degree
of homogeneity of particles which was measured here with the help of a zetasizer. Here we get a value for these formulations 0.35-
0.42 which was within a uniform range of pH was tested for all the formulation
2:8 to 8:2 containing losartan , and the
result was obtained in between 4.60- 6.2. The pH of the optimum formulation
(F5) 5.38±.01, which was present within the range (4.5- 5.5), which makes it
promising for topical delivery [15].
Zeta potential can be measured by
the ability of ionisation of the liquid surface and
it is related with the particle electrokinetic
properties [16]. The stability of these nanoemulsion formulations can be
related with the droplet surface charge, which increase the stability by
electrostatic repulsion, and these can be determine from the zeta potential
value [17]. From the literature we know that the nanoemulsion is stable when
the zeta potential value is greater the 25 mv [18]. In our study, we get the
zeta potential value was -50 mv for the optimum formulation (F5), and the
values for the other samples were shown in the table. With this
characteristics, the permeation of drug improve across the skin occurs through
electrostatic repulsion [19]. Drug entrapment percentage was observed to be
highest in formulation F5, which was 78%, other value were also shown in the
table 2.
Stability studies:
In case of nanoemulsion
development the major problem which demands the maximum attention is the
stability problem [20]. It is intended that a product should be physically and
chemically stable throughout its shelf life period. It has been observed in our
study that polydispersity index droplet size, zeta
potential of the samples did not change significantly through three freeze thaw
cycles. When the nanoemulsion was exposed to centrifugal stress, no evidence of
phase separation and creaming or cracking were observed. For long term
stability studies, it was stored for 180 days in a well-sealed amber colored
container in room temperature and no significant change was observed. It was
found that the nanoemulsion formulation was stable in both stressed conditions
and in a long time frame.
Ex-vivo skin permeation study:
The transdermal permeation
profile of losartan through goat ear skin from nanoemulsion was a typical steady state profile. After
a lag time of 2 h for losartan nanonemulsion, a
nearly linear relationship between cumulative amounts of drugs permeated versus
time could be observed, indicating that the skin integrity was maintained
throughout the experiment and the permeation rates were constants. A low
permeation flux of 1.39 µg/cm2/h demonstrated that the system was ideal for
slow release of losartan.
CONCLUSION
Conventional delivery of losartan
by oral route possesses several drawbacks, such as it has low bioavailability
due to high pass metabolism. Also it suffers from low half-life inside the
biological system. In this present work, the transdermal delivery system of
losartan through nanoemulsions was thoroughly
investigated. Losartan-loaded nanoemulsions for
transdermal delivery, containing linseed oil as the oil phase, Tween 80 as the
surfactant, span 80 as the co-surfactant, were prepared. Stability studies
performed under different stress and temperature conditions showed that the
formulation could be stored for a long period without exhibiting significant changes
of its physicochemical properties. Ex vivo drug permeation studies showed that
losartan permeation across the skin layers occurred at a slow rate while
maintaining integrity of the goat ear skin. These results suggested that on
linseed based nanoemulsion can used as promising carriers for transdermal
delivery of losartan. However, future investigation on in vivo efficacy of
delivered losartan must be performed before introducing the formulation for
clinical applications.
REFERENCES
1. Remington In: The Science and
Practice of Pharmacy, 21st ed.Maryland: Lippincott
Williams & Wilkins; 2007: 1289.
2. M.B. Brown, G.P. Martin, S.A.
Jones, F.K. Akomeah, Dermal and transdermal drug
delivery systems: current and future prospects, Drug Deliv.13 (2006)175–187.
3. H.A.E. Benson, Transdermal
drug delivery: penetration enhancement techniques, Curr.
Drug Deli. 2 (2005) 23–33.
4. S. Khurana
, N.K. Jain , P.M.S. Bedi Nanoemulsion
based gel for transdermal delivery of meloxicam: Physico
-chemical, mechanistic investigation ,life scinces92(2013)383-393.
5. A. Kogan
, N. Garti, Microemulsions as transdermal drug delivery vehicles, Adv.
Drug Deliv. Rev.123 (2006) 369–385.
6. S. Peltola,
P. SaarinenSavolainen, J. Kiesvaara,
T.M. Suhonen, and A. Urtti,
Microemulsions for topical delivery of estradiol,
Int. J. Pharm. 254 (2003) 99–107.
7. F. Shakeel,
W. Ramadan, Colloid Surf., B: Biointerface75 (2010)356–362.
8. De-Hua
He, Jin-Xiu Lin, Liang-Min Zhang, Chang-Sheng Xu, Qiang Xie,Early
treatment with losartan effectively ameliorates hypertensionand
improves vascular remodeling and function in antihypertensive rat model, Life
Sciences(2017) doi: 10.1016/j.lfs.2017.01.013.
9. M.S Shams, M.I Alam, A. Ali, Y. Sultan,
M. Aquil
,et al. Pharmacokinetics of a losartan potassium released from a
transdermal therapeutic system for the treatment of hypertension, Pharmazie 65(2010) 679-682.
10. R. Thaku
, M.K .Anwer ,M.S. Shams, A. Ali, R. K. Khar ,E. I. Taha, Proniosomal transdermal therapeutic system of losartan
potassium: development and pharmacokinetic evaluation, J Drug Target 17( 2009)
442-449.
11. A. Divsalar,
A.A. Saboury, M. Nabiuni,
Z. Zare, M.E. Kefayati, A. Seyedarabi, Colloid Surf. B: Biointerfaces
98 (2012) 80–84.
12. J. Malakar,
A. Basu, A.K. Nayak,
Candesartan Cilexetil Microemulsions
for Transdermal Delivery: Formulation,nin-vitro Skin
Permeation and Stability Assessment,
Current Drug Delivery11(2014) 313-321.
13. R. Gannu,
V. Yamsani, M.R. Yamsani,
Enhancement potential of Aloe vera on permeation of
drugs with diverse lipophilicities across rat
abdominal skin. Curr. Trend Biotechnol.
Pharm. 2(4)(2008) 548-554.
14. P. Fernandez, V. Andre, J. Rieger, A. Kuhnle, Nano-emulsion
formation by emulsion phase inversion, Colloids Surf., A: Physicochem.
Eng.Aspects, 251 (2004) 53–58.
15. H. Wagner, K.H. Kostka, C.M. Lehr, U.F. Schaefer, pH profiles in human skin:influence of two in vitro test systems for drug
delivery testing, Eur. J. Pharm.Biopharm. 55 (2003)
57–65 .
16. V.E. de Campos, E.
Ricci-Junior, C.R. Mansur, Nanoemulsions as delivery
systems for lipophilic drugs, J. Nanosci. Nanotechnol. 12 (2012) 2881–2890.
17. S.S. Lim, M.Y. Baik, E.A. Decker, L. Henson, L.M. Popplewell,
D.J. McClements, S.J. Choi, Stabilization of orange
oil-in-water emulsions: a new role for ester gum as an Ostwald ripening
inhibitor, Food Chem. 128 (2011) 1023-1028.
18. H.A. Lieberman, M.M. Reiger, G.S. Banker, Pharmaceutical dosage forms: disperse
systems, in: S.E. Tabibi (Ed.), Journal of
Pharmaceutical Sciences, Wiley Subscription Services, Inc., A Wiley Company,
New York, 2006, p. 856.
19. P. Becker, Macroemulsions, in: M.J. Schick (Ed.), Nonionic Surfactantes: Physical chemistry, Marcel Dekker, New York,
1987, pp. 435–491.
20. I.B. Pathan,
C.M. Setty, Stability evaluation of tamoxifen citrate nanoemulsion
containing Cremophor RH 40 as surfactant. Acta Pharm. Sci.53( 2011) 127-134.